The objective of a worldwide research initiative in which Würzburg physicist Adriana Pálffy-Buß is participating is to develop a novel and more accurate method for measuring time. The findings may potentially contribute to the investigation of dark matter.
The global navigation system (GNS), the Global Positioning System (GPS), digital data transmission inside the telephone network, and satellite-based earth measurement. The functionality of these devices is contingent upon the presence of accurate timekeepers. In this context, the accuracy of the findings relies heavily on a few billionths of a second. The field of science, particularly physics, relies heavily on very accurate clocks in order to investigate phenomena such as the composition of dark matter or the constancy of natural constants.
The approval of an international research project has provided a fundamentally new foundation for the development of a high-precision watch. The establishment of a “special research area” by the Austrian Science Fund FWF at the conclusion of 2023 might be likened to the establishment of a collaborative research center by the German Research Foundation (DFG).
Teams from the University of Vienna, the Vienna University of Technology, the Institute of Science and Technology Austria, and Julius-Maximilians-Universität Würzburg (JMU) will work together over the next four years. This project has been allocated funding of 3.1 million euros. The project includes the participation of Adriana Pálffy-Buß from Würzburg. The expert in X-ray quantum optics assumed the chair for theoretical quantum information and quantum optics at JMU at the start of 2022. Additionally, she engages in research within the Würzburg-Dresden Cluster of Excellence, specifically focusing on the field of Complexity and Topology in Quantum Materials (ct.qmat).
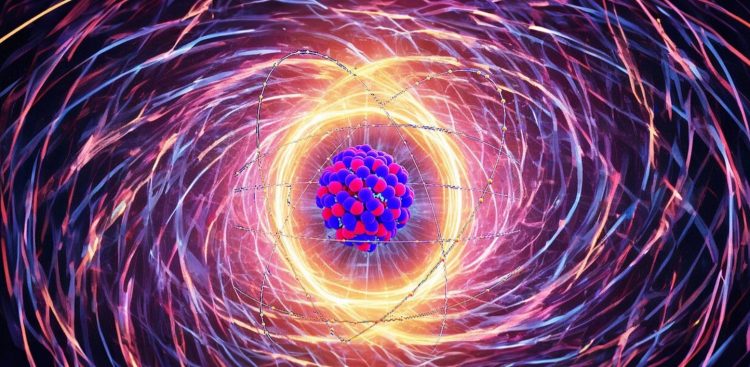
Enhancing the Precision of Measurement in Physical Methods
Oliver Heckl, a researcher affiliated with the University of Vienna, aims to enhance the precision of measurements in the field of coherent metrology beyond electric dipole transitions. The FWF news statement states that a novel technique using light with orbital angular momentum will be used. What is the definition of this?
Atomic clocks are currently the most accurate timekeeping devices available. They measure time by analyzing the frequency of electron transitions between various energy levels inside an atom. Our initiative aims to use a recently created narrow-band laser to induce a transition of an atomic nucleus between energy levels, resulting in the emission of photons, which are particles of light. According to Adriana Pálffy-Buß, the use of a nuclear clock has the potential to enhance measurement accuracy by around three.
Investigation into a specific isotope of the mineral thallium
The primary area of interest for the study team is a specific isotope of the element thorium. The thorium nucleus under consideration consists of 229 nuclear constituents, namely protons and neutrons. It has the ability to attain an excited state that is about eight electron volts higher in energy compared to its lowest energy level, often referred to as the ground state.
“According to Pálffy-Buß, the disparity between these two states is so minute according to the principles of nuclear physics that they were barely distinguishable upon initial observation.” Simultaneously, it is this disparity that has the potential to enable the feasibility of a “nuclear time measurement.” In the year 2023, empirical evidence demonstrated the emission of a photon along with the transition of a thorium nucleus from its excited to ground state.
Utilize a laser to emit thorium atoms and then catch the desired photons. Regrettably, the functionality of the “nuclear clock” is not straightforward. One causal factor for this phenomenon is that approximately eight electron volts are required to stimulate the nucleus. Nevertheless, a magnitude of six electron volts is sufficient to dislodge the outermost electron from its orbital path. In this scenario, the nucleus that is in an excited state has a preference for transferring its excess energy to the electron rather than producing a photon. “Nevertheless, it is imperative to prevent this,” elucidates the scientist.
One potential approach to addressing this issue is the integration of thorium atoms into specialized transparent crystal structures. According to Pálffy-Buß, the studies conducted demonstrated that thorium assumes its position inside the crystal lattice in an ionic form, indicating its relinquishment of its outer electron. The crystal has the ability to accommodate many thorium nuclei simultaneously, facilitating the detection of the desired photon.
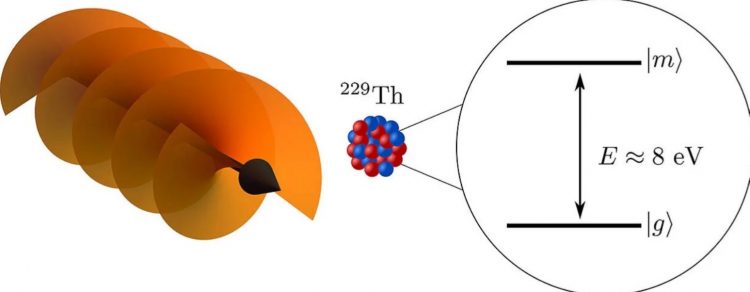
Using rotating corkscrews as a solution
Yet another issue arises: currently, no laser possesses the required level of precision to initiate the intended outcome. The Austrian-German research team is thus placing their trust in the mentioned “cutting-edge technique that harnesses light with orbital angular momentum.” This phenomenon is also known as twisted light or vortex beams.
In simple terms, laser pulses do not collide with the thorium atoms as if they were encountering an impenetrable barrier of energy in this approach. Instead, they have a resemblance to a rotating corkscrew, which increases the likelihood of achieving the desired excited state of the atomic nuclei.
Theoretical calculations for the ideal scenario
Adriana Pálffy-Buß, with her expertise in theoretical physics, will primarily contribute to the research project through her calculations. “I design and simulate the outcomes of different experimental setups and provide recommendations on what would be most effective,” summarizes the physicist. She carefully considers various approaches in order to determine the most promising scenario. She is awarded approximately 375,000 euros from the funding pot of the special research area, which is sufficient to support two doctoral positions.
According to Pálffy-Buß, this research project is incredibly thrilling for physicists. Investigating concepts that are typically assumed to be true, such as the constancy of fundamental physical constants, becomes possible with the advent of a nuclear clock. Additionally, it may provide insights into the composition of dark matter. According to the physicist, the nuclear clock has the ability to provide answers to these questions thanks to the fundamental interactions involved in nuclear transitions.